How Muscles Work (Part 1 of 2)
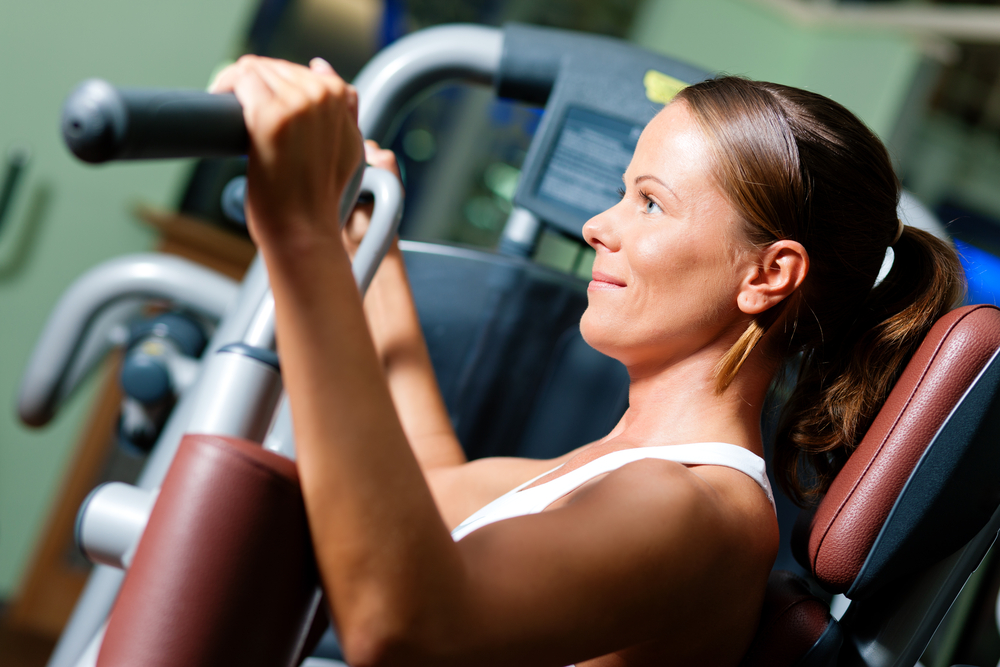
This is the first of a two part series explaining how muscles work. In this first article I describe the gross structure of skeletal muscle; in the second article I describe skeletal muscle ultrastructure and how muscles develop tension.
Gross Structure of Skeletal Muscle
Humans possess three types of muscle—cardiac, smooth, and skeletal—each exhibiting distinct functional and anatomical differences, see below.
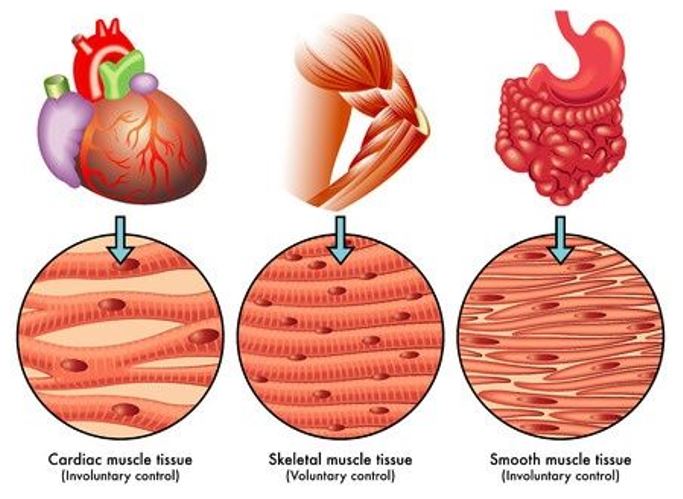
Cardiac muscle resides only in the heart. It shares several common features with skeletal muscle as both appear striated (striped) under low-magnification microscopic examination and both shorten (contract) in a similar manner. Smooth muscle lacks a striated appearance but shares cardiac muscle’s characteristic of nonconscious regulation under autonomic nervous system control. Skeletal muscle operates under voluntary control, as in curling a 25-lb barbell.
Individuals can easily control the velocity of movement in a barbell curl, the range of motion during the lifting movement, and the number of repetitions completed. In golf, the player controls all aspects of the coordinated and hopefully perfectly timed movements of the arms, legs, and torso during the backswing and downswing. A different situation exists for both cardiac and smooth muscle tissue because activity of these tissues occurs involuntarily, although mediation from central centers can exert some influence. This means a general absence of conscious control as to how fast the heart beats, or how fast food moves through the digestive system, or how the miles of blood vessels contract and expand throughout the day.
Skeletal Muscle
The figure below illustrates a cross section of skeletal muscle structures and arrangement of connective tissue wrappings, including the thousands of cylindrical wells called fibers.
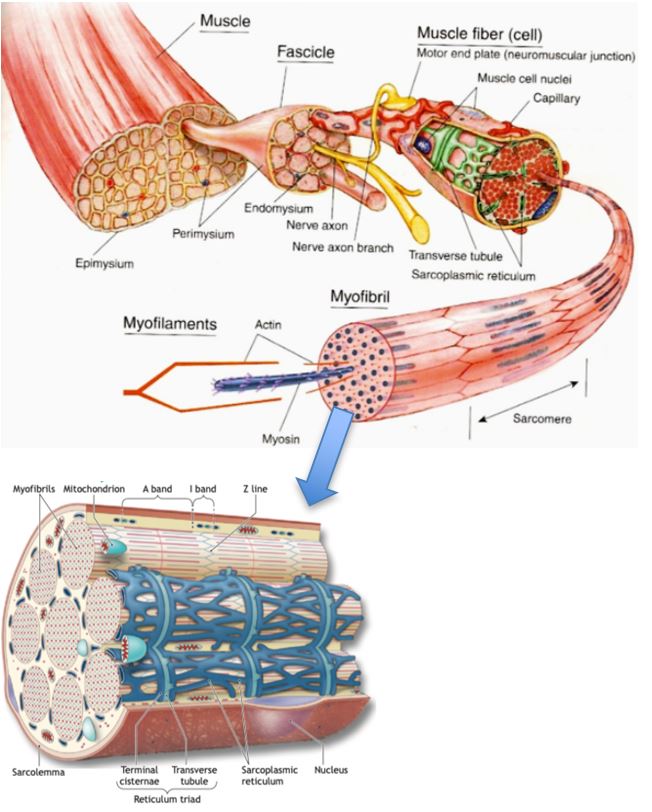
Bottom: Cross section of the sarcoplasmic reticulum and T-tuble system that surrounds the myofibril.
Each of the body’s approximately 600-plus skeletal muscles contain various wrappings of fibrous connective tissue. These long, slender, multinucleated fibers lie parallel to each other, with the force of action directed along the fiber’s long axis. Their number probably remains largely fixed by the second trimester of fetal development. Individual fiber length varies from a few millimeters in the eye muscles to nearly 30 cm in the large antigravity leg muscles (with width reaching 0.15 mm).
Skeletal Muscle Organization
The endomysium, a fine layer of connective tissue, wraps each muscle fiber and separates it from neighboring fibers. Another layer of connective tissue, the perimysium, surrounds a bundle of up to 150 fibers called a fasciculus. A fascia of fibrous connective tissue, the epimysium, surrounds the entire muscle. This protective sheath tapers at its distal and proximal ends as it blends into and joins the intramuscular tissue sheaths to form the tendon’s dense, strong connective tissue. Tendons connect both ends of the muscle to the periosteum, the bone’s outermost covering.
FYI— Tendinitis, a condition of tendon inflammation, most commonly occurs from trauma at the patellar tendon of the knee (common in basketball and volleyball athletes) and other body regions. These include the Achilles region of the ankle (common in sports requiring high impact during lunging and jumping activities), or at the attachment of the rotator cuff muscles, a group of muscles and their tendons that act to stabilize the shoulder (common in sports that involve high-velocity baseball pitching, shotput, or discuss throwing). Tendinitis also can occur from overuse and putting limbs through extreme movements that exceed the joints’ normal range of motion. In less severe tendon trauma, common therapies include nonsteroidal anti-inflammatory medicines (NSAIDs), immobilization, ice, and rest, with gradual return to normal physical activities. |
The tissues of the tendon intermesh with the collagenous fibers within bone. This forms a powerful link between muscle and bone that remains inseparable except during severe stress when the tendon can sever or literally pull away from the bone. When the tendon attaches to the end of a long bone, the bone adapts by enlarging at that end to create a more stable union.
The force of muscle action transmits directly from the connective tissue harness to the tendons, which then pull on the bone at the point of attachment. The forces exerted on the tendinous attachments under muscular exertion range from 20 to 50 N (197 to 492 kg) per cm2 of cross-sectional area—forces often larger than the muscle fibers themselves can tolerate.
The muscle’s origin refers to the location where the tendon joins a relatively stable skeletal part, generally the proximal or fixed end of the lever system or that nearest the body’s midline; the point of distal muscle attachment to the moving bone represents the insertion.
Beneath the endomysium and surrounding each muscle fiber lies the sarcolemma, a thin, elastic membrane that encloses the fiber’s cellular contents. It contains a plasma membrane (plasmalemma) and a basement membrane. Between the basement and plasma membranes lie myogenic stem cells known as satellite cells, these normally quiescent myoblasts function in regenerative cellular growth provide possible adaptations to exercise training and recovery from injury.
Incorporation of satellite cell nuclei into existing muscle fibers seems a likely explanation for exercise-induced muscle fiber hypertrophy. The fiber’s aqueous protoplasm or sarcoplasm contains enzymes, fat and glycogen particles, nuclei (approximately 250 per mm of fiber length) that contain the genes, mitochondria, and other specialized organelles. The sarcoplasmic reticulum, an extensive longitudinal latticelike network of tubular channels and vesicles provides structural integrity to the cell.
Muscles’ Chemical Composition
Water constitutes approximately 75% of skeletal muscle mass while protein composes 20%. The remaining 5% contains salts and other substances, including high-energy phosphates; urea; lactate; the minerals calcium, magnesium, and phosphorus; various enzymes; sodium, potassium, and chloride ions; and amino acids, fats, and carbohydrates. The most abundant muscle proteins include titin, the largest protein in the body consisting of 27,000 amino acids (accounts for about 10% of muscle mass), myosin (approximately 60% of muscle protein), actin, and tropomyosin. Each 100 g of muscle tissue contains about 700 mg of the oxygen-binding, conjugated protein myoglobin.
Muscles’ Blood Composition
Arteries and veins that lie parallel to individual muscle fibers provide a rich vascular supply. These vessels divide into numerous arterioles, capillaries, and venules to form a diffuse network in and around the endomysium. Extensive branching of blood vessels ensures each muscle fiber an adequate oxygenated blood supply from the arterial system and rapid removal of carbon dioxide in the venous circulation. During vigorous physical activity for an elite endurance athlete, the muscle’s oxygen uptake increases nearly 70 times to approximately 11 mL per 100 g per minute. The local vascular bed delivers large quantities of blood through active tissues to accommodate this oxygen requirement. Blood flow distribution fluctuates in rhythmic running, swimming, cycling, and other similar activities. Flow decreases during the muscle’s contraction phase and increases during relaxation to provide an auxiliary “milking action” that moves blood through the muscles and propels it via the venous system back to the heart. Between 200 and 500 capillaries deliver blood to each square millimeter of active muscle cross section, with up to four capillaries directly contacting each fiber. In endurance athletes, five to seven capillaries surround each fiber; this positive adaptation ensures greater local blood flow and adequate tissue oxygenation when needed.
Physical activities that require “straining” (i.e., exerting force against an immovable object) present a somewhat different picture for muscle blood flow. When a muscle generates about 60% of its force-generating capacity for several seconds, elevated intramuscular pressure occludes local blood flow during the contraction. With a sustained high-force contraction, the intramuscular high-energy phosphates and glycolytic anaerobic reactions provide the main energy source for muscular effort.
Muscles’ Capillarization
Trained muscles’ increased capillary-to-muscle fiber ratio helps to explain improved exercise capacity with endurance training. An enhanced capillary microcirculation expedites removal of heat and metabolic byproducts from active tissues in addition to facilitating delivery of oxygen, nutrients, and hormones. Electron microscopy reveals the total number of capillaries per muscle and capillaries per mm2 of muscle tissue averages about 40% higher in endurance-trained athletes than untrained counterparts. Enhanced vascularization at the capillary level proves particularly beneficial during activities that require a high level of steady-rate aerobic metabolism. Vascular stretch and shear stress on the vessel walls from increased blood flow during exercise stimulate capillary development with intense aerobic training.
Source: McArdle WD, Katch FI, Katch VL. Exercise Physiology: Nutrition Energy, and Human Performance. Eighth Edition. Wolters Kluwer Publ. 2015.